3D Modelling of Underground Excavations with EX3 and RS3
The principal objective of this article is to outline how the 3D numerical modelling software, RS3, based on the finite element method (FEM), and EX3, the recently released overhaul of Examine3D, complement each other in the analysis and design of underground excavations for civil engineering and mining applications. The article targets practicing geotechnical engineers and engineering geologists who need to model underground excavations. Many of these professionals possess a firm understanding of geotechnical engineering, particularly the physical behaviour of geological materials. What many of them lack is highly specialized numerical modelling skills. Practicing geotechnical engineers are also typically constrained by tight project timelines and limited budgets; they often need to obtain answer questions over a short time.
The relatively simple, inexpensive, and fast-computing EX3, can be used by practitioners to study the effects of excavation on stresses and displacements of geological material and major geological discontinuities such as faults. EX3 is well suited for elastic analysis involving large extents and irregular geometries (which is particularly true of mining). Parts of the discussion will detail the types of problems that EX3 can analyze and the program’s advantages and limitations. The article will also describe the power of RS3 and how that can be combined with EX3 to facilitate good geotechnical engineering.
The article is laid out as follows:
- First introduces readers to underground geomechanics problems and why numerical modelling tools are essential for problem-solving in everyday engineering of underground excavations
- Provides a brief overview of numerical methods for underground excavation analysis, shows the classes to which RS3 and EX3 belong, and the programs’ advantages and limitations
- Discusses the problems caused by excavating near or across major geological structures such as faults
- Outlines types of underground excavation analysis and design problems that EX3 and RS3 can address, and insights that engineers can gain using both software, and
- Summarizes the attractiveness of both software.
Numerical Methods for Geomechanics
Different types of soil and rock failure mechanisms, ranging from the simple to the very complex, can occur with underground excavation under the combined influence of geology, in situ stress and mining/geotechnical conditions (including excavation geometries and layout). It is best to study these problems with numerical modelling software tools.
The purpose of all numerical modelling in rock engineering is to analyze the behaviour of geological media and structures under excavation and other loading conditions. Numerical methods aim at producing ‘acceptably accurate’ solutions to complex geomechanics problems by dividing domains into equivalent systems of smaller, interconnected elements and determining approximate solutions. By necessity, models incompletely represent the real world. However, they allow us to investigate the responses and characteristics of geotechnical structures/excavations and anticipate how they will behave under different conditions.
Numerical methods attempt to satisfy several theoretical geotechnical requirements, including
- Realistic material stress-strain responses and their related parameters
- Boundary conditions that realistically simulate real-world conditions
- Excavation geometry and layouts
- Construction sequence
- Interactions between support and surrounding ground, and
- Consideration of pore water pressures
With recent advances in computing, numerical methods are enjoying increasing use in the solution of three-dimensional problems.
Numerical modelling can help engineers and engineering geologists gain valuable insights into potential failure modes and areas in which these are likely to occur. This aspect enables geotechnical professionals to develop designs and solutions. Numerical modelling tools help geotechnical professionals rationally compare design alternatives and identify those requiring more detailed analysis. (This is one of the critical ways in which EX3 and RS3 complement each other and help engineers optimize the use of time and budgetary resources.)
Numerical tools are particularly useful for undertaking parametric and sensitivity analyses. They help engineers and geologists appreciate the uncertainty and risks associated with design choices.
The numerical methods can be classified into the following three main categories:
- Continuum methods
- Discontinuum methods, and
- Hybrid continuum/discontinuum methods.
Continuum methods assume that the continuity of all points in a problem domain is always preserved. As a result, even when discretized into elements, these methods ensure continuity between the elements. Discontinuum methods treat individual elements as being separate but interacting with each other. Whereas continuum methods focus on the deformations of a system, discontinuous methods typically target the rigid body motions (usually large movements) of individual elements.
The most popular continuum methods are the Finite Element Method (FEM), Finite Difference Method (FDM) and Boundary Element Method (BEM). The most widely used discontinuum methods are the Discrete Element Method (DEM) and Discontinuous Deformation Analysis (DDA).
The FEM is the numerical method most widely applied to a wide range of problems in soil and rock. It can accommodate geometries of geotechnical structures, complex loading paths, and nonlinear behaviour of soils and rocks, among many other important aspects. However, models are typically larger, require more computing power and take longer to compute. RS3 is a full 3D FEM implementation.
As the name suggests, the boundary element method (BEM) ‘discretizes’ (or divides into elements) the boundaries in a problem domain. Boundaries include geometric entities such as excavation surfaces, discontinuities, and material interfaces, for multi-material problems. Because the BEM discretizes only boundaries, model sizes are typically much smaller and compute times faster. However, it is not as generalized as the FEM. EX3 is a BEM program.
The next sub-section will briefly describe key features of the BEM and FEM.
The Boundary Element Method
There are two types of BEMs – direct boundary element methods and indirect boundary element methods. The direct boundary element method solves directly for unknown stresses and/or displacements from specified boundary conditions. Direct boundary element methods are constrained to simple problems such as half space problems in civil engineering.
The indirect boundary element method uses fictitious stresses on boundaries to first calculate the stress conditions on the boundaries and then applies separate relationships to find boundary displacements. For underground excavations, the indirect boundary element method (fictitious stress method) is widely used. 3D (Examine 3D, Curran & Corkum, 1993) and 2D (Examine 2D, Curran & Corkum, 1994) boundary element programs were used in this research.
Under the class of indirect BEMs, there is a method known as the Displacement Discontinuity Method (DDM). It is well suited to the modelling of tabular orebodies, excavations, and major geological structures such as faults.
With most numerical methods, far-field stress and displacement conditions must be approximated in some way. This is typically done by truncating the problem domain at some distance beyond the zone of influence of the excavation and fixing the outer boundaries. The BEM captures excavation influence into infinity, i.e. far-field stresses are not influenced by the creation of excavations. Consequently, for problems involving linear elastic, homogeneous material domains, the BEM offers a major advantage of correctly modelling far-field boundary conditions, and confining discretization errors to problem boundaries. It ensures that stresses and displacements vary continuously throughout the problem domain, and problems compute much more rapidly than other numerical methods.
The ability to inherently model far-field stress conditions can be disadvantageous in the solution of problems involving different geological units of finite extents. For such problems, the BEM requires special numerical techniques. These are implemented in EX3 and allow the program to be applied to multi-material problems.
The Finite Element Method
The FEM originated in the early 1940s from the solution of complex elasticity and structural analysis problems in civil and aeronautical engineering. The FEM develops systems of algebraic equations that approximate solutions for partial differential equations. It accomplishes this by subdividing a problem domain into smaller, simpler components – elements.
The FEM can be applied to a very broad range of geotechnical engineering problems that require calculation of stresses, displacements, pore pressures and forces in support elements. In many cases, what limits the FEM’s application are factors such as computational resources, time, acquisition of appropriate input data and not inherent limitations of the method. The method can account for complex in situ stress conditions, linear and nonlinear stress-strain behaviour, multiple materials and support systems, excavation and construction sequences, groundwater conditions, and mix of boundary conditions, among other factors.
3D Numerical Modelling
In the past, when there were no easy-to-use three-dimensional analysis tools, engineers simplified all problems to two dimensions. Sometimes they did so using questionable assumptions. Today, the availability of software tools such as EX3 and RS3, truly three-dimensional aspects, such as excavation layout and sequencing, can be readily modelled. These software tools can also model the localized and mine-wide influence of major geological structures (such as faults, shear zones and dykes) on excavations. For example, numerical modelling software can help engineers and geologists to determine excavation conditions under which faults can slip and sometimes induce seismic events.
Input Parameters for Numerical Modelling
From the above discussions, inputs for numerical analysis of underground excavations include the following:
- In situ stress states (principal stress magnitudes and orientation)
- Excavation geometry, layout and sequence
- Strength and deformation characteristics of rock mass units, faults and other large-scale geological structures
- Strength, deformation and mode of action of support systems or elements
- Location and orientation of faults relative to excavations (whether they daylight/intersect or are simply situated close to excavations)
- Number of faults, and
- Water pressures
RS3 takes all of the above into account. EX3 accounts for most except for pore pressures and the influence of structural support elements.
What are the effects of faults on underground excavations?
It is not uncommon for orebodies to occur within or near geological discontinuities such as faults, shear zones and intrusions. Civil engineering infrastructure such as power caverns and tunnels also regularly encounter major geological structures. When major discontinuities lie within rock mass volumes influenced by excavation, stress redistributions can strongly depart from classic continuum mechanics and must be studied closely. Modelling of the interactions between excavations and faults, particularly at mine-wide scale, is a key challenge in the geomechanics of underground excavations. As a result, this section of the article will discuss details of these interactions.
Slip and separation of geological structures that daylight into excavations can generate stress relaxation, a major cause of excavation instability. In mining, for example, relaxation occurs in hanging walls. Relaxation typically allows the formation of gravity-induced failures (falls of ground) and significantly influences ore dilution. Faults also act as free surfaces that help create rock wedges and facilitate wedge movements. Differences in the strength and deformation characteristics between fault materials and adjacent rock mass units also contribute to the redistribution of stresses and displacements with excavation.
When a fault does not intersect an excavation but lies close to it, slip along the fault can concentrate stresses in the rock mass volume between the excavation and the fault. In high stress environments (such as those encountered in deep mining or tunnelling through high mountains), this stress concentration can lead to rockbursts.
It can be seen from the above discussions that the degree to which faults influence excavation stability is determined by excavation geometry, locations of faults relative to excavations, in situ stresses, and the strength and deformation characteristics of the rock mass units and faults.
RS3 and EX3 can efficiently model excavation-fault interactions. EX3 is the preferred tool when trying to understand the influence of mine-wide excavations on fault behaviour due to the method’s use of surface discretization only. RS3, on the other hand, is better suited to understanding the details of excavation-fault interactions, resulting redistributions and the design of support for such areas.
Combination of EX3 and RS3 in Practice
EX3 combines the DDM with the conventional indirect BEM. As a result, although EX3 models only elastic material behaviour, it can capture the inelastic effects of separation and slip of large-scale discontinuities on stress redistributions around excavations. If such analysis with EX3 indicates zones of inelastic response that are relatively large compared to the dimensions of excavations, it may be necessary to undertake more detailed studies into these effects using RS3. However, even in such cases, surprisingly useful engineering insights can be established using the simpler approaches of EX3.
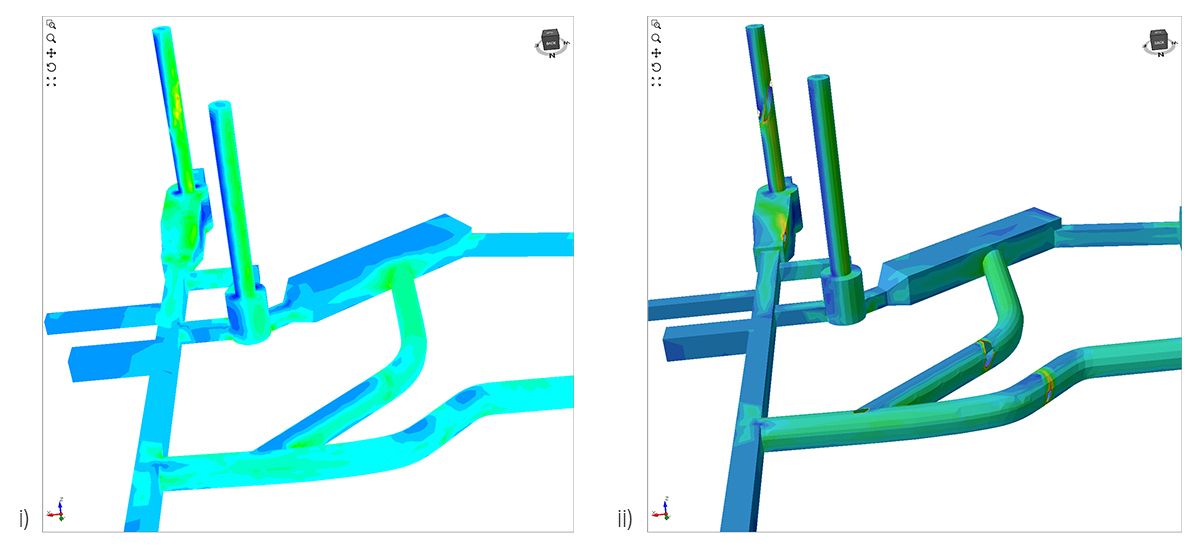
For the above reasons, in large-scale problems Rocscience recommends that EX3 elastic analysis precede more sophisticated RS3 analysis. EX3 allows for quick, inexpensive exploration of the effects of varying design controls such as excavation dimensions, shape, location, orientation and sequence. Once key understanding has been gained, RS3 can be deployed to model inelastic material behaviour, groundwater effects, and to design support elements such as bolts, liners.
For smaller scale excavations such as those encountered in civil engineering, both EX3 and RS3 can again be used to assess the stability of rock masses immediately adjacent to excavations, including when fault intersect the excavation or pass close by the excavation. Typical examples are found in the excavation of tunnels and power caverns. Again, EX3 offers the advantage of rapid computing of simple elastic material response. The simple-to-use EX3 elastic stress-strain analysis tool can be used to estimate fault-slip potential of faults. EX3 can be used to estimate the location and size of excavation disturbance zone based on in situ stresses, strength and deformation properties of rock masses and discontinuities, and the layout and geometries of excavations.
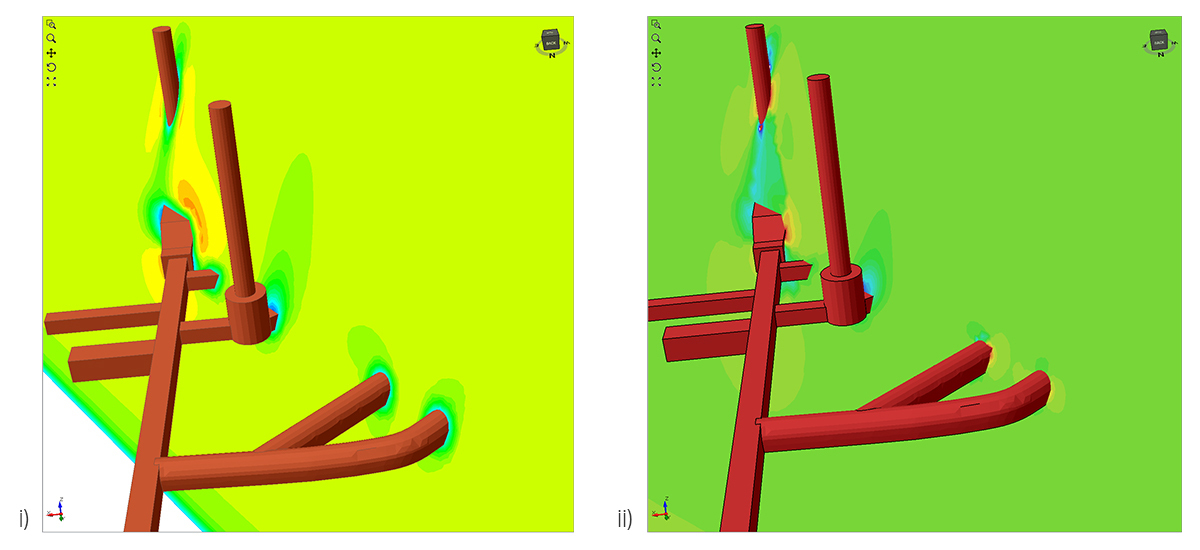
Elastic analysis based on a linear relationship between stress and strain ignores peak and post-peak strength behaviour and can significantly overestimate calculated stresses, strains and kinetic energies. RS3 can be employed to evaluate the ‘true’ magnitudes of stresses and extents of failure zones. It can also be used to assess the interactions between support elements and the ground being supported.
Both EX3 and RS3 can be powerful in assessing potential pillar behaviour and evaluating different strategies and tactics (such as backfill support) for minimizing negative impacts of stress redistribution from excavation.
Concluding Remarks
Considerable understanding into the behaviour of rock masses and major geological structures due to excavation (both mining and civil) can be gained using numerical modelling tools, including EX3 and RS3. EX3 combines speed and simplicity, making it useful for generating preliminary insights into stress problems, and analyzing large-scale underground mines and tunnels. It is very helpful in studying interactions between different excavations, and between excavations and geological structures. RS3, on the other hand, is more powerful and versatile in handling a broad range of geotechnical inputs and problems, including complex material, soil-structure interactions and support behaviours.
Although EX3 and RS3 do not in themselves solve problems, they offer the tools for tackling your practical, engineering challenges. The two software help you to manage the challenge of appropriately balancing accuracy with costs such as available time, computational resources and budgetary constraints.