What Cappadocia’s Soft Rocks Teach Us About Underground Storage Caverns
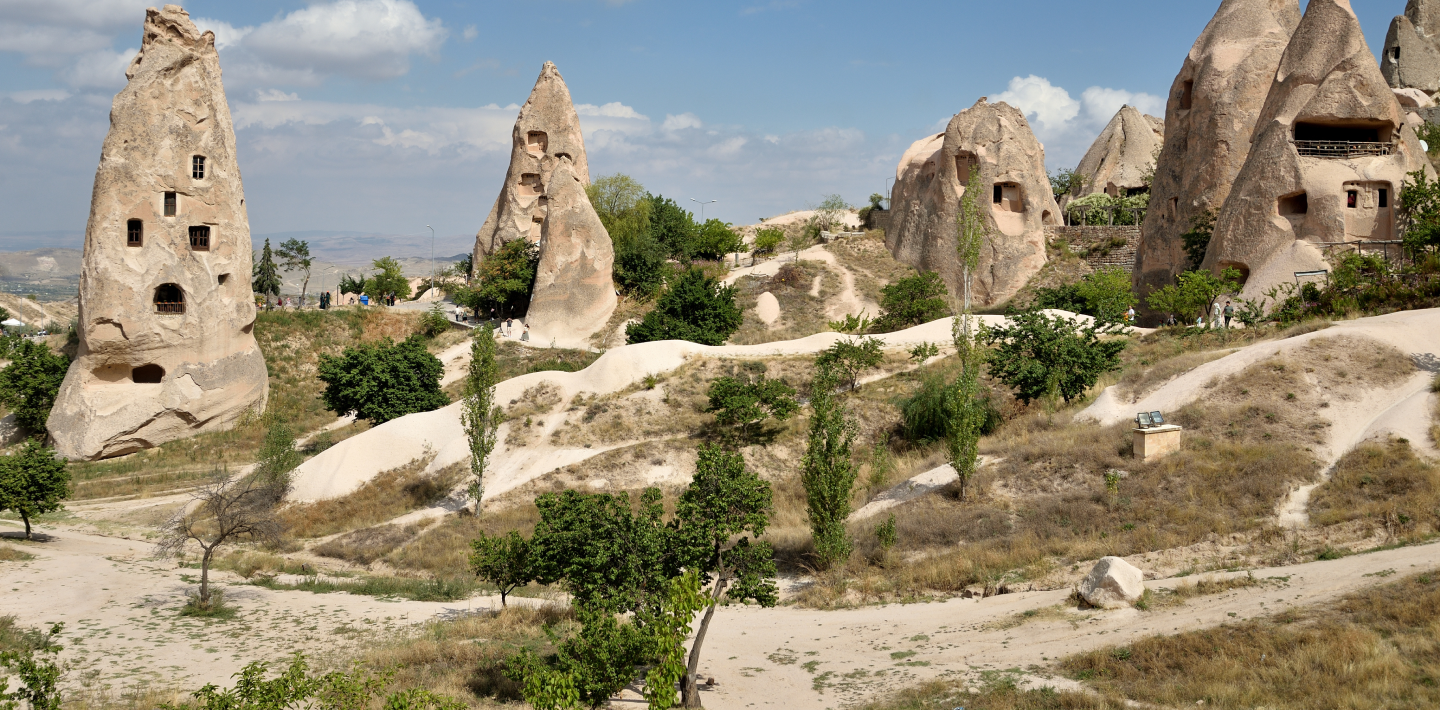
Beneath the famous fairy chimneys of Cappadocia, Türkiye lies a vast network of underground caverns carved into soft tuff rock — a fragile material. There’s a growing need for cost- and energy-efficient storage solutions for agricultural products in the area, so the demand for underground storage caverns is rising despite the complicated geology.
Traditional two-dimensional analysis methods alone can fail to capture the cave network’s true behavior, which can lead to overly conservative designs or unanticipated risks. As a result, Dr. Mehmet Sari turned to RS2 and RS3, our advanced finite element modelling tools, to address these challenges. His research shows that by integrating 2D and 3D analysis, engineering teams can better optimize design parameters, minimize risks, and meet stringent safety standards without unnecessary costs.
In this case study, we’ll cover how RS2 and RS3 contributed to the project, and for further details and relevant figures, read Dr. Sari’s research paper.
The Geological Setting
The volcanic tuff formations of Cappadocia are layered with ignimbrites, pumices, and porous sediments, which are easy to carve but are less predictable because they’re prone to weathering and deformation. Geological strength index (GSI) values in the region range widely, from 55 in the fractured, discontinuous masses to 100 in the homogeneous intact rock.
Underground storage caverns can be used for agricultural storage to take advantage of the tuff’s thermal properties and maintain stable indoor temperatures. However, ensuring their stability is difficult due to the rock’s variability and vulnerability to failure mechanisms like roof collapse and pillar instability.
The Research Challenge
First, because the soft tuff rock exhibits highly variable geological properties, predictions of deformation and stability were complicated. Second, the caverns’ complex geometry — with interacting galleries, rooms, and pillars — introduced intricate stress patterns that traditional analysis methods can struggle to capture.
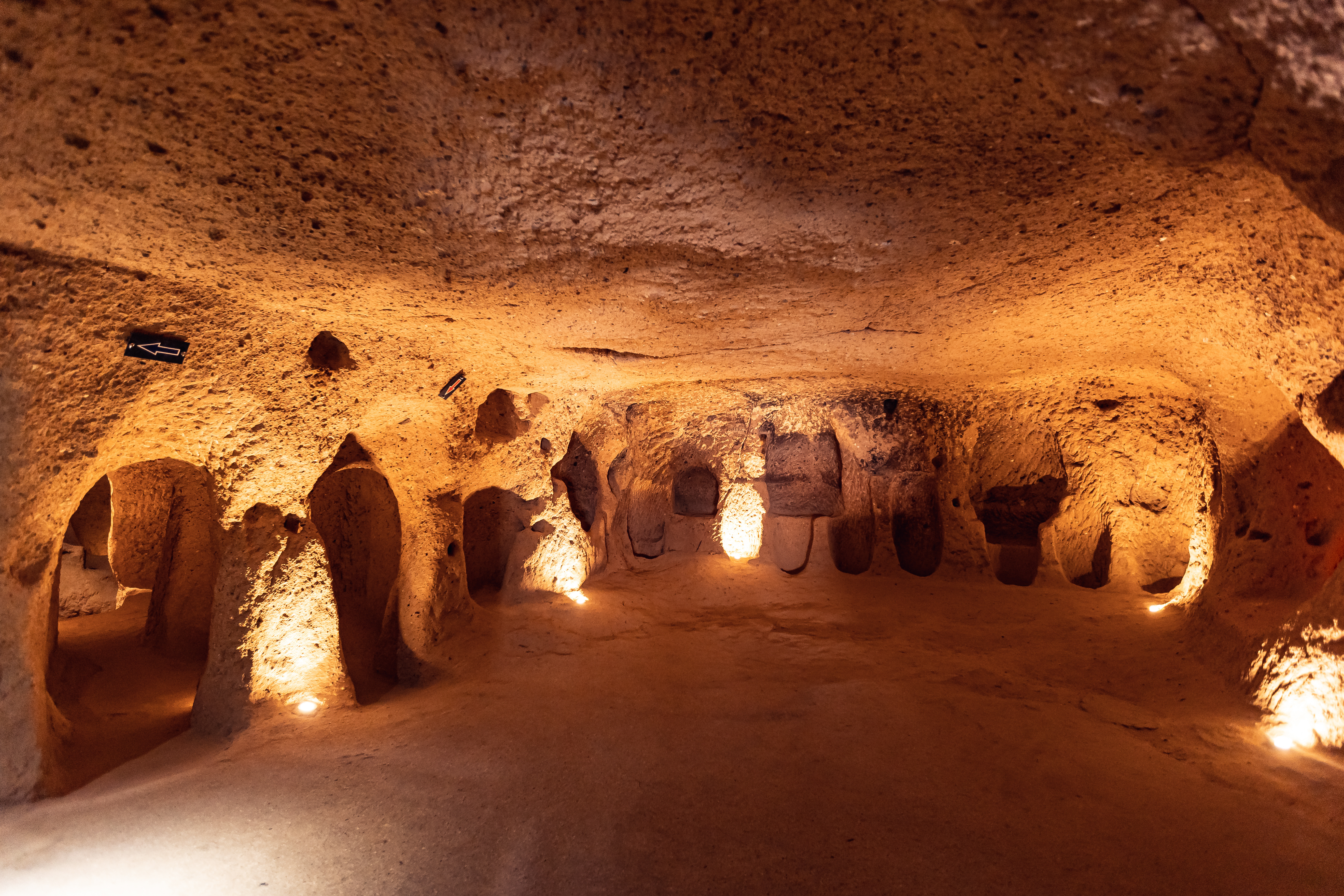
To meet regional safety standards, the cavern needed to achieve a factor of safety (FoS) of at least 1.5 under static conditions. However, early-stage analyses often overestimated failure risks or overlooked critical interactions within the structure. Reliable modelling tools were needed to optimize the design and ensure stability.
The Solution
Dr. Sari used RS2 and RS3 for a comprehensive analysis of cavern stability in the research. RS2 was employed for 2D modelling of transversal and longitudinal cross-sections, which provided initial insights into stress distribution and deformation in specific sections. While valuable for identifying potential failure points, its 2D scope could not fully capture the caverns' complexity.
RS3 was then used for modelling the entire structure in 3D. By accounting for the interactions between rooms, galleries, and pillars, RS3 gave a realistic assessment of stress distributions and failure mechanisms. Two geological scenarios were tested — one with intact rock (GSI=100) and one with fractured rock (GSI=55) to account for real-world variability in rock mass conditions. Both models explored a range of cover depths, from 5 to 20 meters, to determine optimal design parameters.
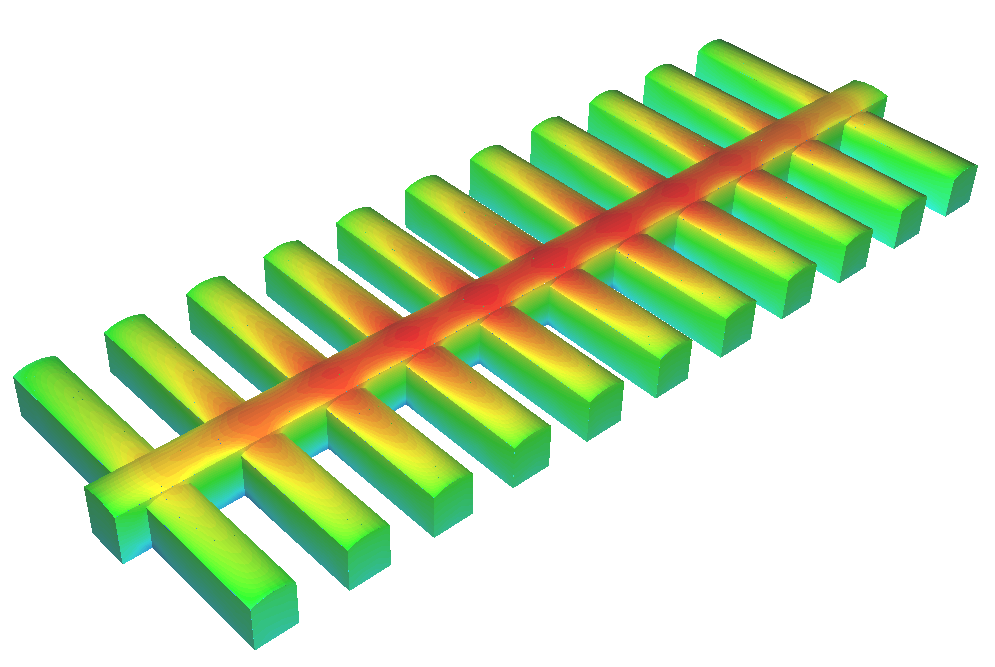
See figures 6-9 in the original research paper to see the contours of total displacement and maximum shear strain in a typical underground storage cavern’s longitudinal cross-section of RS2’s analysis. See figures 13-14 for the total displacement and maximum shear strain contours on the excavation boundary, based on RS3 results.
The Results
RS2 and RS3 combined yielded actionable insights that improved the design process. The 2D analysis in RS2 revealed potential instability points like roof collapses in the main gallery and shear strain concentrations at the intersections between rooms and pillars. The 3D analysis in RS3 offered a more accurate representation of failure mechanisms by incorporating the effects of complex geometries. For example, maximum displacements were observed at the top-center of the main gallery, while maximum shear strains occurred along the storage room boundaries nearest to the gallery.
The ideal cover depth was determined to be 15 meters, as it minimized deformation and maximized stability. In best-case conditions (GSI=100), RS3 calculated a high FoS of 12.14. Even in worst-case conditions (GSI=55), the FoS reached 1.63, exceeding the minimum safety threshold.
RS3's analysis also revealed stress interactions across multiple rooms, with total displacement counters showing concentrated deformations in the middle rooms and the central main gallery at deeper cover depths.
The Verdict
In Cappadocia’s challenging geotechnical environment, RS2 and RS3 helped engineers understand how to better design underground storage caverns in soft tuff rock. By examining varying rock mass parameters and analyzing 2D and 3D geometric effects, the research uncovered a clear relationship between safety factors and the caverns’ structural design. With the two programs together, engineers can better tackle geological uncertainty, optimize designs, and ensure compliance with strict safety standards.
What RS2 and RS3 Brought to the Project:
- Reliable modelling: RS3 provided a reliable understanding of stress distributions and failure mechanisms for the cavern’s 3D geometry, which helped the team account for interactions between rooms, galleries, and pillars that traditional 2D methods could not alone address.
- Efficient use of resources: By identifying areas where deformation risks were minimal, RS3 can help engineers use reinforcements strategically, reducing unnecessary material costs while maintaining safety standards.
- Improved safety assessments: RS3 and RS2 together helped the engineers predict and address potential failure mechanisms, such as roof deformations and pillar stress concentrations. This can help maintain long-term stability for underground structures in challenging geologies.
Frequently Asked Questions
What is an underground storage cavern?
An underground storage cavern is a stable and environmentally controlled space that’s typically carved out of rock formations like limestone, salt, or soft volcanic tuff. These caverns have natural insulation and can maintain the consistent temperatures and humidity levels necessary to preserve perishable products.
They’re often used to store goods and materials, and the industrial and energy sectors use them to store oil, natural gas, or compressed air for energy production. The rock’s geology plays a role in the cavern’s design and functionality, as their safety is influenced by factors like stability, thermal conductivity, and porosity.
What are common failure mechanisms in underground caverns?
Roof collapse, pillar instability, and stress propagation are all common failure mechanisms. Roof collapses can occur from insufficient cover depth or poor rock quality, which leads to excessive deformation or tensile failure at the cavern’s crown. Pillar instability – where inadequately spaced or undersized pillars can result in overstressing – leads to progressive failure or cascading collapses.
Stress propagation between rooms or galleries is particularly significant in soft or discontinuous rock masses. Stress concentration in one area can redistribute forces to neighboring sections, amplifying deformation and triggering failure in interconnected areas.
What materials are best for reinforcing underground caverns?
The right reinforcement materials to use depend on the geology and failure risks of the cavern. For roof stability, rock bolts and shotcrete provide immediate support and prevent detachment of rock blocks. In areas requiring higher load-bearing capacity, try steel ribs or lattice girders. Anchors and cable bolts are often used to stabilize large structures or deep excavations.
In soft rock, mesh and fiber-reinforced shotcrete are particularly effective, as they provide flexibility and strength to withstand deformation. For pillar reinforcement, grout injections can strengthen weak zones by filling fractures and increasing cohesion. Overall, your material choice should be based on detailed geotechnical assessments and compatibility with the host rock and expected loads.
How can thermal properties of rock influence underground storage?
For rocks like Cappadocia’s tuff, with high porosity and insulation properties, it’s easier to maintain stable indoor temperatures year-round. Because there’s less of a need for artificial climate control, these caverns are ideal for storing goods like vegetables, fruits, and dairy products for a lower cost. Stable thermal conditions can also prevent damage to sensitive items such as chemicals or electronics and minimize energy costs for refrigeration or heating. Poorly insulated or thermally conductive rock may require more intensive climate control measures.
What are the long-term challenges of maintaining underground caverns?
Weathering, groundwater intrusion, and structural degradation are common challenges for maintaining underground caverns. Exposure to air and moisture leads to gradual rock mass weakening for soft or porous rocks, and groundwater intrusion accelerates this process in regions with fluctuating water tables or poor drainage systems.
Examples of structural degradation over time include joint widening, roof sagging, and pillar erosion, which all can compromise stability. To detect signs of these instabilities early, you can regularly monitor with deformation meters and laser scanning. Reapplying shotcrete, reinforcing pillars, or grouting fractures are solid strategies to maintain caverns.